Mitigation of water logging and salinity through biodrainage: potential and practice
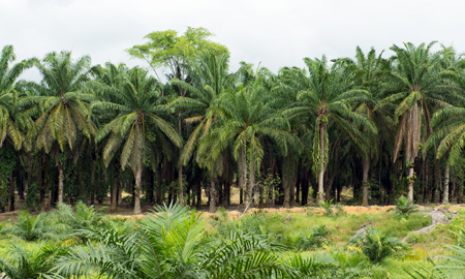
Summary
Over the past hundred years, vast areas worldwide have been used for intensive agriculture following clearance by removal of deep rooted tree vegetation or by introducing irrigation in arid zones.
After decades of profitable returns, many of these domains, particularly those underlain with saline aquifers and with poor natural drainage have degraded owing to water logging and salinity.
Disturbed hydrological balance in the form of sustained percolation of surplus surface rain or irrigation waters to the saline water table resulted in waterlogged and saline conditions.
Surface and sub-surface drainage can be an effective remedy, but has limited applications in marginal farm lands. During the last two decades, there has been awareness of the potential for biodrainage to remove surplus soil water.
This is typically effected by forming a water table depression down slope of a tree plantation or discharge area that may extend up to several meters (around) beyond the plantation.
This lowers the water table below the root zone of the (surrounding) crop area. Biodrainage has low establishment costs and no effluent disposal problems.
Biodrainage systems have been successfully tested worldwide, including India, and a strong case for their large scale adoption can be made.
The authors opine a paradigm shift in the approach of policy makers and drainage engineers in recognizing the role of trees as potent drainage modules. Sensitization of the affected farming communities to adopt locally suited biodrainage based agroforestry models is also desired.
Keywords
Biodrainage, salinity, water logging, water table, socio-economical, policies.
Glossary
An aquifer is an underground layer of water-bearing (water saturated) area from which groundwater can be extracted using water well.
Aquifers having dissolved salts are not fit for irrigation or for potable water purposes.
Biodrainage is the vertical drainage of water table through evapo-transpiration of strategically planted vegetation, particularly deep rooted trees. Frequent use of the term ‘biodrainage’ in scientific literature has been only post 2000.
Agricultural drainage is a system of open channels, subterranean pipes through which the water level on or in the soil of a cropping area is controlled.
Rio summit is the United Nations Conference on Environment and Development (UNCED) held in Rio de Janeiro from 3 to 14 June, 1992.
Salinity is the excessive content of salts (generally chlorides and sulphates of sodium, calcium and magnesium) in soil- or irrigation-water. Salinity is easily measured by measuring electrical conductivity of water. A conductivity value greater than 4000 Siemens per second is harmful for most crops.
Water logging of soil agriculturally is said to occur when the water table of the groundwater is too high, so that it saturates the soil in the crop root zone, resulting in prolonged anoxic conditions.
Introduction
Background:
Over the past hundred years or so, countries throughout the world have introduced intensive agriculture on various pristine domains of nature, each having its own peculiar ecology.
In many cases, after a few decades profits have declined and such interventions have not remained sustainable. In this paper our primary concern is the vast area of in arid and semi-arid land where deeper layers of soil had ancient stores of salts.
Here agriculture-led disturbed hydrological balance has resulted in the percolation of water with a gradual rise in salinity in the crop root zone ultimately leading to development of salt pans and water logging. These changes in irrigated lowlands due to intensive agriculture has become critical so that certain areas are non-cultivable (1,2,3).
It is a matter of common knowledge that an engineering drainage system is needed in such situations to maintain a check on the salinity-water table menace, but our paper deals with the non-conventional drainage system i.e. biodrainage which has caught the attention of workers throughout the world.
We shall confine our discussion mainly to the role of trees in drainage of the water from our saturated top soils of agricultural use. We shall also briefly describe the role of trees in areas where groundwater tables have declined to abnormally low depths. We shall also suggest some policies which should be adopted.
Soil water use by trees and biodrainage:
Trees can transpire large amounts of water, for example, Euperua purpurea in Amazonian rainforest was estimated to transpire 1180 kg/ day of water (4).
Equally noteworthy is the ecological level interaction of deep rooted trees with groundwater. Thus, as early as 1953, Wilde et al. (5) noted that tree species influence the groundwater table by acting as biological pumps. FAO (6) highlights the positive and negative effects of trees like Eucalyptus on groundwater.
However, large scale scientific use of trees in ground water control seems to be of more recent origin. The concept of biological drainage or biodrainage appears to have originated in the waterlogged agricultural areas where the conventional surface and sub-surface drainage techniques were in vogue (7).
Biodrainage may be defined as the vertical drainage of water through evapo-transpiration of strategically planted vegetation, particularly deep rooted trees with the intention of lowering the water table.
Conventional drainage and biodrainage
Success and limitations of conventional drainage:
Conventionally the control of water logging and soil salinity has been obtained through civil engineering techniques such as surface drainage, horizontal sub-surface drainage and vertical drainage (8,9,10).
Surface drainage excavation of open trenches is done to drain surface water and to prevent pond formation, flooding and consequent damage to the crops.
In the horizontal sub-surface drainage removal of soil water below the crop root zone is done through a network open tile drains. A better option is to install a network of perforated subterranean pipes.
In either case water or dissolved salts leach into the tile drains or pipes preventing both water logging and salinity. In the vertical drainage system bore wells are dug and the water is pumped out.
If not saline, this water can be used for irrigation or pumped into the adjoining canals to augment flow. In semi-arid zones, where groundwater is saline, a conjunctive use helps in irrigation and prevents a gradual rise in the water table.
However, these techniques, particularly horizontal sub-surface drainage, are costly to install, maintain and sustain (1,2,11).
They also have the problem of effluent control. If discharged into natural drains the saline effluent pollutes the rivers downstream. If reused, even conjunctively, salts are redistributed in the agro-ecosystem and the problem of salinity increases over a period of time.
Ritzema et al.(2) opined that in developing countries like India fragmented landholdings of marginal farmers are not suitable for the adoption of these techniques as compared to more developed countries, where agriculture is carried out on an industrial scale.
Advantages and disadvantages of biodrainage:
Biodrainage is an ecologically attractive concept which has the merits low cost and environmental friendliness.
The limitations are a requirement of land for tree plantations, slow and uncontrolled lowering of water table, limited evacuation of salts from the system, and vulnerability of trees to highly saline conditions.
Recharge and discharge zones:
In planning a biodrainage system the need for recharge and discharge zones should be clearly understood.
Recharge areas are locations from where water seeps into the water table, e.g. leaky canals or tributaries and elevated areas receiving rainfall with runoff water. However, the most significant recharge areas are the agricultural fields where liberal canal irrigation is applied.
The areas where biodrainage plantations are raised to offset the recharge water are known as discharge areas. On average about 10% of land in a waterlogged agricultural landscape is to be marked as a discharge area.
Biodrainage and representative problem areas
Perusal of the literature shows that two unique situations exist where large scale field level biodrainage efforts have been made. These are outlined below:
Clearing of deep rooted vegetation in high rainfall zones:
In Australia the pristine deep rooted tree and heath vegetation overlaid brackish water aquifers and ancient stores of salt.
This was because the annual rainfall was intercepted and evapo-transpired by the native vegetation. Introduction of intensive agriculture necessitated the clearing of this tree vegetation and its replacement with shallow rooted annual crop plants.
The annual water consumption by this vegetation was less than the rainfall and as result water percolated to the underlying saline groundwater table causing its gradual rise.
The twin menace of salinity and water logging appeared.
Now suitable development of agroforestry systems incorporating trees are expected to reduce the salinity as the water table recedes from the root zone of commercially important annual crops (13,14,15,16).
This model of ‘ecosystem mimicry’ (17) intends to obtain a plant-water use scenario that closely imitates the pre-clearing situation. The Australian system is the most exhaustively studied disturbed agro-ecosystem that unambiguously demonstrates the necessity of harmony between water use by vegetation and aquifers (Fig. 1).
Introduction of irrigation in arid and semi-arid zones:
In semi-arid north west India, the traditional rain fed agriculture was not affected by the deep underlying saline groundwater.
The introduction of canal irrigation and intensive agriculture upset this balance. Gradual seepage of the liberally used irrigation water caused a rise of the saline water table so that soils became waterlogged and saline.
For example, in the western zone of Haryana, average water table depth was static at about 28 m from the ground surface between the 1930s and early 1950s.
Since the commissioning of the Bhakhra canal system in 1956, the water table rose to only 6m from the ground surface by 2002 (Fig. 2, 3, 4).
During the past two decades nearly 50% of the area of south-west Haryana has been critically waterlogged with the water table rising to <3 m of the ground surface during this time (1).
The phenomenon is worldwide, but biodrainage systems are being considered and integrated with the existing agro-ecosystems for example, in China (18), India (12,19,20,21), Israel (22), Pakistan (23) and Uzbekistan (24,25).
Impact of biodrainage on depression of water table
Biodrainage certainly depresses the water table immediately underneath plantations, but in an agroforestry the objective is to lower the water table to a safer depth, well below the crop root zone in the cultivated area that surrounds the plantation (7,12).
A cone of water table depression with the lowest point near the cavity of a well is known to develop as a result of pumping from the aquifer (unconfined aquifer).
Further, if two wells are operating simultaneously at a suitable distance, two ‘interfering’ cones of depression will be formed.
The draw down effect of two related Eucalyptus tereticornis block plantations was similar to the combined interacting cones of depression of two pumping wells (20).
Biodrainage and soil salinity
Remediation of soils waterlogged with (fresh) water is a less common phenomenon as fresh water can be readily utilized for both agricultural and non-agricultural purposes.
A more threatening situation arises where water logging involves a saline aquifer or a soil profile with an ancient store of salts. In either case interaction of a biodrainage plantation with the saline waterlogged soil becomes inevitable.
It is well established that physiologically most of the trees growing under saline conditions exclude salts, especially Na+ and Cl- which are excluded by the root and do not form a part of the transpiration stream.
Theoretically, therefore, these may accumulate under the plantation over a period of time. This may result in a buildup of salinity in the root zone and pose a risk to the survival of the biodrainage system itself.
In Australia, a 7 year old Eucalyptus plantation surrounded by an irrigated area there was a significant lowering of the water table beneath the plantation or discharge area but no accumulation of salt with respect to the outside irrigated recharge area.
However, after 15 years accumulation of salts had taken place in the capillary fringe above the water table areas (14) of this plantation.
Archibald et al. (26) examined the sustainability of Eucalyptus plantations on saline discharge areas and concluded that although soil salinity develops beneath the plantations, there was an excellent survival of plantations even after 20-25 years.
Biodrainage strategies
Pit versus ridge planting:
Soils waterlogged up to surface or sub-surface zones are anaerobic and the conventional pit planting technique is not feasible.
On such problematic locations soil ridges raised up to 0.5 m above the surrounding soil surface is recommended (20).
This aids the better establishment and subsequent growth of seedlings on waterlogged soils as it enables them to withstand anaerobic conditions produced by prolonged water logging or ponding.
The ridge planting technique is being successfully practiced by Punjab and Haryana State Forest Departments in India. Since ridges are made from the field soil, they have the same salinity.
Salt tolerant species are therefore, recommended. Eucalyptus, Pongamia, Casuarina, Terminalia, etc can grow while poplars and bamboos could not survive (Table 1).
To avoid excess of salts accumulation due to surface evaporation, ridges can be covered with sand to discourage capillary fringe.
Block plantations:
A block of suitable trees is planted in a waterlogged area, which causes a cone of water table depression underneath the plantation.
However, the extent of lowering of the groundwater table around the surrounding recharge area has been shown to vary from a radius of 40 m (15) to 730m (20).
The vast differences may be attributed to the size and other characteristics of the discharge plantation block, hydraulic conductivity of the soil and cropping pattern, recharge of the surroundings. In the planning of Australian biodrainage systems the plantation discharge areas are confined to saline or degraded areas so that less arable land is lost (26).
Strip plantations
Block plantations work well, but are not feasible where land holdings are small and fragmented where land cannot be spared for biodrainage.
Here strip plantations on field boundaries are the only alternative. In many Indian states, including Haryana, the standard unit of land with field boundaries on all four sides is an acre (0.4 ha) of about 66m length in the east-west direction and 60m width in north-south direction.
Therefore a ‘farmer’s model’ comprising parallel strip biodrainage plantations in a north-south direction 66 m apart and with two rows of trees on each strip raised 0.5 m are recommended (27).
This agroforestry model has been successfully tested on a pilot scale around a village (Putthi) in the district of Hisar in Haryana.
The model is considered as best option from the point of view of: i) technological adoption by the farming community, ii) lowering of the water table to about 1 m over a period of 5 years and iii) remuneration to the farmers as timber (27).
The authors (28) compared the biodrainage potential of ten tree species planted as per this farmer’s model (Fig. 5, 6).
It was revealed that about five years old trees differed significantly in their biodrainage potential in the order: Eucalyptus tereticornis clone 10 = Eucalyptus hybrid (clone of E. tereticornis x E. camaldulensis) > Eucalyptus clone–130 = Tamarix aphylla > Prosopis juliflora > Eucalyptus clone–3 > Callistemon lanceolatus = Melia azedarach > Terminalia arjuna = Pongamia pinnata.
There are several pockets of land where water logging is so acute during the post-monsoon months of end-October to December that the top soil is saturated water so that field preparation/sowing of wheat is not possible.
It is on such locations that the transfer of raised field bund technology for biodrainage is adopted even by marginal farmers without much questioning.
Future scope and conclusions
Research and development
International research efforts on biodrainage have been coordinated by an International Program for Technology and Research in Irrigation and Drainage (IPTRID) of the FAO of the UN (7).
In India the Indian National Committee on Irrigation and Drainage (INCID), operating under the Ministry of Water Resources (MoWR), Government of India, coordinates national level biodrainage research funding and knowledge synthesis (29).
Adoption constraints:
The conduits of surface, sub-surface and vertical drainage are essentially civil engineering structures and biodrainage may be a difficult concept for civil engineers to consider.
Farmers may also be wary of any negative effects tree plantations may have with their crops. This feeling emerged at a National Level Training Program comprising senior irrigation engineers, agricultural scientists and foresters facilitated by the authors (30).
However, once aware of the issues these personnel could see the feasibility and advantages of integrated conventional and biodrainage.
Biodrainage cannot be effective where acute and prolonged flooding, or ponding conditions, prevail. Here only surface drainage can be effective.
However, where over the years a rise in the water table is a threat, properly designed biodrainage can replace or complement subsurface and vertical drainage. In future there may be increasing exploitation of trees for better groundwater hydrology, agroforestry, forestry, urban development and a green and clean environment at large.
Creation of livelihood:
Widespread adoption of biodrainage is likely to depend upon use of trees with alternative uses, for example, of poplars on alluvial plains of north-western India, have improved the income of farmers (Fig.7).
This is due to the fact that a well developed marketing system is available. Eucalypts can make a similar impact on waterlogged soils as they are well adapted to waterlogged conditions.
Productivity and profitability of plantations of Eucalyptus have been revolutionized with the development of genetically improved fast growing and high yielding clonal planting material.
Average productivity of commercial clones is about 20-25 m3/ ha/ yr. Where World Bank aided forestry development projects existed, many States in India have adopted clonal plantations of Eucalyptus.
This has been very helpful to the farmers. It is important to note that there is no self propagation of eucalypts in India.
Prosopis juliflora and Prosopis pallida, are excellent biodrainers (21), which can be used for plantations on waterlogged soils.
Many of the problems of Prosopis are a result of using unsuitable strains of P. juliflora. Seeds from Peruvian material assumed to be P. pallida is superior to material of P. juliflora available in India.
Prosopis pods are high in sugars, carbohydrates and protein and can be used to prepare animal cakes.
It fruits annually and crops evenly in an adverse climate. P. juliflora produces wood with a high calorific value of approximately 21 MJ/kg (5000 kcal/kg), so that charcoal obtained from the wood of Prosopis species is of very high quality.
Ten kg of green wood will make 1-2 kg of charcoal using traditional earth kilns, in 2-4 days. Above ground biomass from different sites varies from as little as 0.5 t/ha/yr to over 39 t/ha/yr.
Frequent cutting produces small branches which are ideal for cooking, so that it provides two important and integral components for rural communities in in some parts of India, especially Chambal Valley (Fig. 8 shows transport of Prosopis juliflora near the Chambal River).
P. juliflora has immense potential on water logged soils and promises to boost the economy of poor rural people It is grown to reduce erosion in hilly areas with unstable soils prone to high run-off.
Casuarina in coastal belts and bamboos in high rainfall areas, are other very important industrial species that can be used for waterlogged conditions.
In order to boost the economy of farmers, there needs to be close collaboration with the companies processing and marketing the timber.
Environmental concerns:
Trees are a valuable carbon sink. They play a vital role in nutrient cycling and of restoring soil fertility, arresting soil erosion and creating a micro-climate suitable for micro- flora and micro-fauna.
The permanent tree cover protects soil from erosion and regulates the water balance. Further, trees and shrubs are less sensitive to fertility levels than food crops and some species help to stabilize degraded land.
Socio-economic concerns:
Apart from the biological and environmental roles, trees also have a significant social, religious and cultural status.
Since the Rio Summit in 1992, global environmental concerns have been acknowledged as integral components of sustainable development.
Planting of trees on wastelands or agricultural lands for industrial and environmental health has been initiated particularly in developing countries.
For example, in Sri Lanka perennial crop-based farming systems supply over 50% of national timber and 80% of fuel wood needs.
Climate change: The rural poor in developing countries, are most at risk of adverse effects of climate change. Biodrainage/agroforestry plantations have the potential to create synergies between efforts to mitigate climate change and efforts to help poor farmers from the adverse effects of climate change.
Fuel wood: A large proportion of the fuel wood for domestic energy in rural areas is harvested from the debris of agriculture and from trees growing outside the forest especially for small landholders. It is therefore, vital to plant trees which provide quality fuel wood whenever possible.
Fodder: Trees play an important role in livestock production since they provide shade, shelter and fodder. Acacia, Prosopis, Leucaena, Albizia, Bauhinia, Celtis, and Grewia are some genera of immense value.
Non-wood products: Wild fruits, herbs, gums, resins, etc. are abundantly produced by improved tree based systems providing diverse products which are linked to socio-economic aspects of society.
Women’s role: In rural economies of developing nations women are more knowledgeable and skillful to handle some operations such as collecting fuel wood, lopping fodder, collecting wild fruits and other non-timber tree products. There are examples, of whole tree based systems, for example, where home gardens are maintained by the women. The tools required, matching their strength need to be developed, and some training to this group can enhance the profit of the family.
Policies
For the effective adoption of biodrainage agroforestry in the farming community particularly in developing countries the following policy points should serve as guide lines:
To detect the places where waterlogging is expected, or has already been revealed and to identify the affected farming community.
* Organize trainings for rural communities that are required to achieve the goal.
* Promote local-level processing and marketing of timber and non-timber tree products relevant to the scale of their production.
* Integrated development plans should include agroforestry/ biodrainage plantations for wood based industries and should promote market demand for farm grown timber.
* Strengthening of farmer groups technically and making available superior tree planting stock for the farmers. Incentives should be provided to farmers growing plantations as trees afford carbon sequestration.
* Site specific R&D is required.
* Knowledge-based adaptive plans should be prepared as per the guidelines of World Agroforestry Centre and National Organizations/Institutions.
References
1. Kumar, R (2004) Groundwater status and management strategies in Haryana. In: Groundwater use in North-west India. (ed., I P Abrol, B R Sharma & G S Sekhon), Centre for Advancement of Sustainable Agriculture, New Delhi, pp 16-26.
2. Quershi, A S, McCornick, P G, Qadir, M & Aslam, Z (2008) Managing salinity and waterlogging in the Indus Basin of Pakistan. Agricultural Water Management, 95, 1-10.
3. Aleksandrova, M, Lamers, P A, Martius, C & Tischbein B (2014) Rural vulnerability to environmental change in the irrigated lowlands of Central Asia and options for policy makers: A review. Environmental Science and Policy (in press).
4. Jordan, C F & Kline, J K (1977) Transpiration of trees in a tropical rain forest. Journal of Applied Ecology, 14, 853-60.
5. Wilde, S A, Steinbrenner R S, Pierce, R S, Dozen, R C & Pronin, D T (1953) Influence of forest cover on the state of groundwater table. Proceedings Soil Science Society of America, 17, 65-7.
6. Poore, M E D & Fries C (1985) The ecological effects of Eucalypts. Rome, Food and Agriculture Organization of the United Nations, Forestry paper No. 59, pp 1- 97.
7. Heuperman, A F, Kapoor, A S & Denecke, H W (2002) Biodrainage – Principles, Experiences and Applications. Knowledge Synthesis Report No. 6. International Programme for Technology and Research in Irrigation and Drainage (IPTRID), IPTRID Secretariat, Rome, Food and Agriculture Organization of the United Nations, pp 1-79.
8. Tanji, K K (1996) Agricultural salinity assessment and management, New York, American Society of Civil Engineers, 1996, ISBN 9 78 078 4473634.
9. Garg, B K & Gupta, I C (1997) Saline wastelands environment and plant growth, Jodhpur, Scientific Publishers,1997, ISBN 9 78 817 2331584.
10. Ritzema, H P, Satyanarayana, T V, Raman, S and Boonstra, J. (2008) Subsurface drainage to combat waterlogging and salinity in irrigated lands in India: Lessons learned in farmer’s fields. Agricultural Water Management, 95, 179-89.
11. Rao, K V G K, Sharma, S K & Kumbhare, P S (2005) Drainage requirements of alluvial soils of Haryana. In: Reclamation and Management of Waterlogged Saline Soils, (ed., K V G K Rao, M C Agarwal, O P Singh & R J Oosterbaan), Central Soil Salinity Research Institute, Karnal and CCS Haryana Agricultural University, Hisar, pp 36-49.
12. Kapoor, A S (2001) Biodrainage – A biological option for controlling waterlogging and salinity. New Delhi, Tata McGraw-Hill Publishing Co. Ltd. 2001, ISBN 9 78 007 0402317.
13. Heuperman, A F (1995) Salt and water dynamics beneath a tree plantation growing on a shallow water table. Internal Report Department of Agriculture, Energy and Minerals, Victoria, Tatura Centre, Institute of Sustainable Irrigated Agriculture.
14. Lafroy, E C & Stirzaker, R J (1999) Agroforestry for water management in the cropping zone of southern Australia. Agroforestry Systems, 45, 277-302.
15. RIRDC (1999) The ways trees use water. Water and salinity issues in Agroforestry No. 5, Publication No. 99/37, Wembley, Rural Industries Research and Development Corporation (RIRDC), pp 1-78.
16. Crosbie, R S, Wilson B, Hughes, J D, McCulloch, C & King, W M (2008) A comparison of the water use of tree belts and pasture in recharge and discharge zones in a saline catchment in the central west of NSW, Australia. Agricultural Water Management, 95, 211-23.
17. Hatton, T J & Nulsen, R A (1999) Towards achieving functional ecosystem mimicry with respect to water cycling to southern Australian agriculture. Agroforestry Systems, 45, 1-3.
18. Zhao, C, Wang, Y, Song, Y & Li, B ( 2004) Biological drainage characteristics of alkalized desert soils in north-western China. Journal of Arid Environments, 56, 1-9.
19. Angrish, R , Toky, O P & Datta, K S ( 2006) Biological water management: Biodrainage. Current Science, 90: 897.
20. Ram, J, Garg, V K, Toky, O P, Minhas, P S, Tomar, O S, Dagar, J C & Kamra, S K (2007) Biodrainage potential of Eucalyptus tereticornis for reclamation of shallow water table areas in north-west India. Agroforestry Systems, 69, 147-65.
21. Toky, O P, Angrish, R, Datta, K S, Arora, V, Rani, C, Vasudevan, P & Harris, P J C (2011) Biodrainage for preventing waterlogging and concomitant wood yields in arid agro-ecosystems in North-Western India. Journal of Scientific and Industrial Research, 70: 639-644.
22. Gafni, A & Zohar, Y (2001) Sodicity, conventional drainage and biodrainage in Isreal. Australian Journal of Soil Research, 39, 1269-78.
23. Chaudhary, M R, Chaudhary, M A & Subhani, K M (2000) Biological control of waterlogging and impact on soil and environment. In: Proceedings Eighth ICID International Drainage Workshop, New Delhi. Vol. II,pp 209-22.
24. Khamzina, A, Lamers, J P A, Martius, C, Worbes, M & Vlek, P L G (2006) Potential of nine multipurpose tree species to reduce saline groundwater tables in the lower Amu Darya River region of Uzbekistan. Agroforestery Systems, 68, 151-56.
25. Khamzina, A. Lamers, J P A, Worbes, M, Botman, E, & Vlek, P L G (2006) Assessing the potential of trees for afforestation of degraded landscapes in the Aral Sea Basin of Uzbekistan. Agroforestry Systems, 66, 129-41.
26. Archibald, R D, Harper, R J, Fox, J E D & Silberstein, R P. (2006) Tree performance and
root zone salt accumulation in three dry land Australian plantations. Agroforestry Systems, 66, 191-204.
27. Ram, J (2009) Biodrainage potential of Eucalyptus for the reclamation of water logged
areas. Ph D. Thesis, Nanital India, Kumaon University.
28. Rani, C. Toky, O P, Datta, K S, Kumar, M, Arora, V, Madaan, S, Sharma, P K. & Angrish, R (2010) Physiological behaviour vis-à-vis waterlogging conditions in some tree species. Indian Journal of Plant Physiology, 15, 44-53.
29. Anon 2003. Biodrainage: status in India and other countries. New Delhi, Indian National Committee on Irrigation and Drainage (INCID), pp 1-47.
30. Angrish, R, Toky, O P & Patel, R K (2008) Biodrainage: potential and practice. National Level Training Program, Training Report. Command Area Development and Water Management (CADWM) wing, Ministry of Water Resources, Government of India, New Delhi, 1-6 February, 2008, pp 1-5.
Download pdfFigures
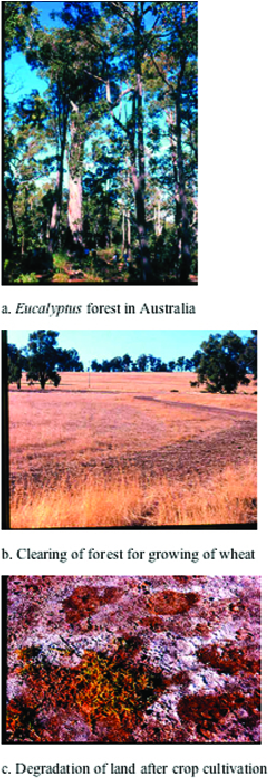
Figure 1. Salinization of land after clearing the forests of Eucalyptus for the purpose of agriculture in Australia.
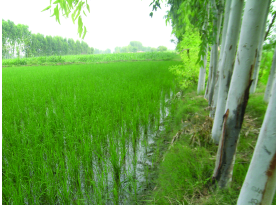
Figure 2. Eucalypts (Eucalyptus tereticornis) biodrainage system on the boundareis in a high water table area of Rohtak district of Haryana State in north India. Paddy is the main crop during monsoon season.
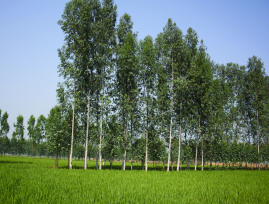
Figure 3. Eucalypts (Eucalyptus tereticornis) biodrainage on the bunds in high water table area of Hisar district of Haryana in north India. Wheat is the main crop during winter.
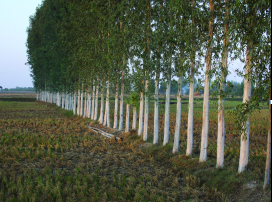
Figure 4. A close view of Eucalyptus tereticornis plantation on the boundaries as explained in figure 3.
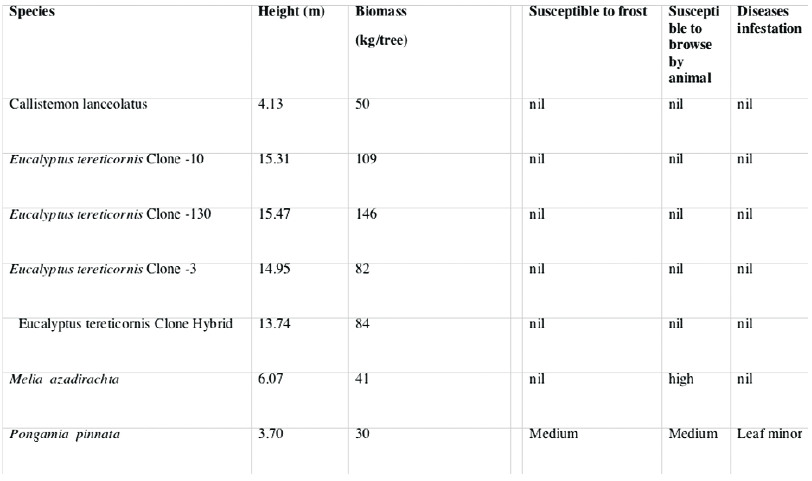
Table 1. Survival and growth of 8-year old trees in a ‘farmer’s model’ (comprising of parallel strip biodrainage plantations in north-south direction 66 m apart and with two rows of trees on each strip on about 0.5 m raised ridges) developed in an area having acute water logging at the campus of Haryana Agricultural University , Hisar, Haryana State in north western India.
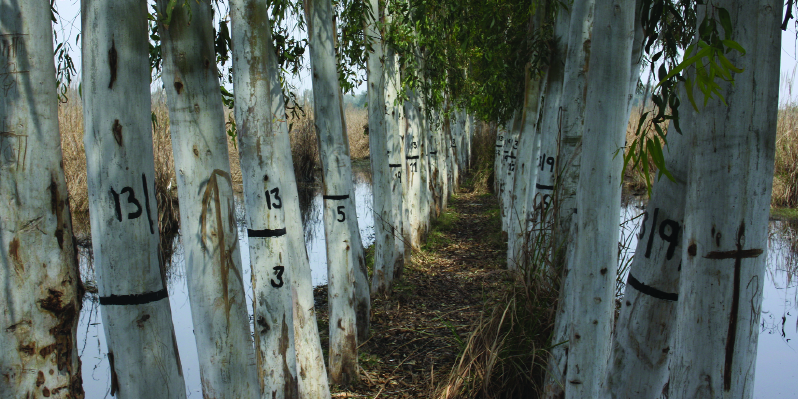
Figure 5. Farmers model of biodrainage developed in a waterlogged area of CCS Haryana Agricultural University at Hisar, north India. Two rows of Eucalyptus tereticornis are raised on the boundareis of the field.
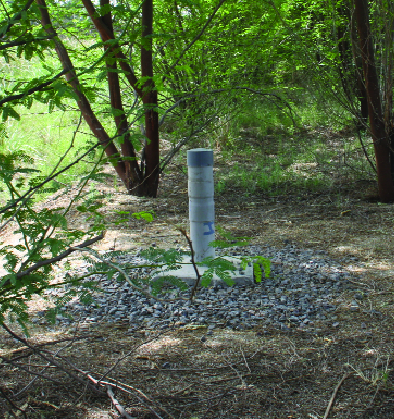
Figure 6. Farmers model of biodrainage developed in a waterlogged area of CCS Haryana Agricultural University at Hisar, north India. Two rows of mesquite (Prosopis juliflora) are raised on the boundareis of the field. The vertical column in the centre of four trees on a two rowed field bund is the observation well for periodic measurement of water table.
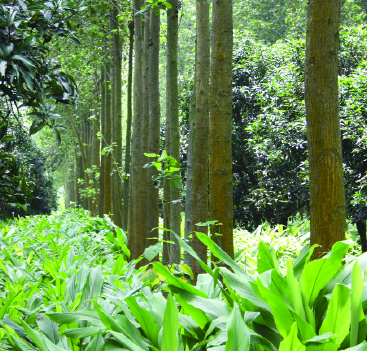
Figure 7. Block plantation of poplars (Populus deltoides) in Yamunanagar district of Haryana, north India. The water table is high and the aquifer has sweet water due to a canal passing nearby. Wheat, sugarcane and fodder crops are grown in the interspaces of poplar trees.
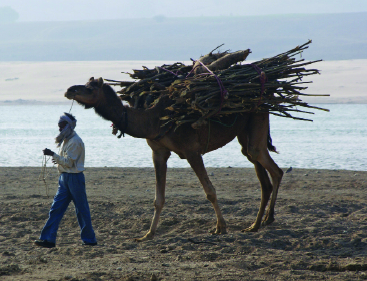
Figure 8. Prosopis juliflora provides firewood for rural communities in some parts of central India. The illustration shows firewood transport along the Chambal River, about 80 km South East of Agra. This plant has been used to stabilize the sandy soils along the river and wood is transported across the river, creating a local industry. (Photo courtesy Mrs Penny Cook)