What role for GM crops in world agriculture?
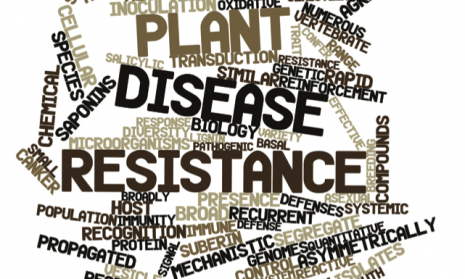
Summary
A critical analysis of claims that genetically modified (GM) crops will play a central role in world agriculture is provided, in the context of current attempts to expand the market for GM seeds in developing countries.
It is argued that smallholder farmers and consumers in developing countries should have more say about R&D investments in order to avoid the opportunity costs associated with misallocation of resources.
Keywords: Genetically modified crops; food policy; agriculture policy; R&D policy
Introduction
Whilst some authors have argued that planting GM crops will be necessary to feed a growing global population, others have argued that this claim has no scientific support, but is rather a reflection of corporate interests, led by the world’s three largest companies utilizing plant gene technology, Monsanto, Dupont and Syngenta, which now control nearly 70% of global seed sales (Jacobsen et al., 2013).
US agricultural biotechnology company Monsanto’s attempts to position GM crops as a “pro-poor” technology are not new (Glover, 2010).
However, there has been a new emphasis in the media on GM crops as the solution to world hunger following the 2008 global food crisis (Stone & Glover, 2011).
Claims that GM crops and foods will benefit poor people in developing countries merit re-investigation, in the light of the significant resources currently being invested in attempts to expand the market.
This critical review first considers the debate regarding current generation GM crops, already on the market, followed by the issues raised by the promised next-generation of GM crops.
Current generation GM crops
Significant investment in GM crop research began in the United States, following the US Supreme Court’s 1980 ruling that genetically-modified organisms (GMOs) were patentable and a report by the US Office of Technology Assessment, which identified GM crops as an area that might deliver a variety of new agricultural traits (OTA 1981).
In 1987, a US National Academy of Sciences report identified investment in biotechnology as the key to agricultural innovation and future competitiveness (NAS 1987).
Despite decades of investment by both the public and private sector, commercially grown GM crops remain largely restricted to the two main traits, herbicide tolerance and insect resistance (Bt crops), in four main staple crops: soy, maize (corn), oil seed rape (canola) and cotton.
More recently, herbicide-tolerant GM sugar beet has also been adopted in the USA. Herbicide tolerant (HT) crops are sprayed with one or more herbicides, which kill weeds but not the GM crop, the largest market share are crops resistant to glyphosate (marketed by Monsanto under the brand name RoundUp).
Bt crops use sequences of genes from the soil bacterium Bacillus thuringiensis (Bt) to express one or more crystal proteins (known as ‘Cry toxins’ or ‘Bt toxins’) which are toxic to some pests.
The three staple GM food crops are grown primarily in North and South America for use in animal feed and industrial scale biofuels (agrofuels), whereas GM cotton is the main crop planted on a commercial scale in India and China.
In the year to 31st August 2011, US- government-subsidised biofuels for the first time overtook animal feed as the main use of domestically-grown maize (Meyer 2011).
US farmers adopted herbicide-tolerant GM crops because of the simplified herbicide regime associated with these crops, however the spread of herbicide-resistant weeds in North and South America (Sanderman 2006, Binimelis et al. 2009) is now impacting significantly on weed management difficulties and costs and resulting in increased use of glyphosate and other herbicides (Bonny 2011; Benbrook 2012).
Pests resistant to Bt crops, and increases in secondary pests unaffected by the Bt toxins in these plants, are also beginning to impact on pest management. Researchers have observed field-evolved resistance to Bt crops by corn rootworm (Gassman et al. 2011); stem borer (Van Rensburg 2007); cotton bollworm (Gunning et al. 2005; Tabashnik et al. 2008) and pink bollworm in the India and China (Dhurua & Gujar 2011; Wan et al. 2012).
In China, initial economic benefits in terms of savings in insecticide use with Bt cotton have been eroded as secondary pests emerged (Wang et al. 2008; Lu et al. 2010; Zhao et al. 2011) and in the USA, western bean cutworm has become an increasingly important pest on Bt corn (Catangui & Berg 2006).
Resistance also develops to pesticides in conventional farming systems, resulting in a “pesticide treadmill”, in which resistant pests encourage applications of larger amounts of current pesticides or the substitution of more toxic pesticides; and a similar “seed treadmill” has been described in which farmers are increasingly locked in to purchasing these inputs from off the farm, rather than saving their own seeds (Howard 2009).
GM seed prices are significantly higher than for non-GM seeds and, in the USA, seed prices have increased significantly as a percentage of operating costs (Benbrook 2012; Bonny 2011).
The benefits to smallholder farmers of adopting crops such as Bt cotton are already strongly contested (Stone, 2012) although pest resistance to Bt cotton is in its early stages.
A major concern is that smallholder farmers could be at risk of being locked into a ‘poverty trap’ by GM seed price hikes and the need for increasing amounts of herbicides and pesticides to tackle weed and pest resistance and shifts in pest populations. Binimelis et al. (2009) refer to this process in Argentina as a ‘transgenic treadmill’.
The prevention of seed saving by patenting and licensing agreements is another major issue for poorer farmers (Oguamanam 2007).
From a commercial developer’s perspective, self-reproducing seeds bypass the profits that could be realized if farmers continued to buy these inputs year after year.
Legal strategies to protect intellectual property rights (IPRs) evolved from protections to certain seeds (e.g., International Union for the Protection of New Varieties of Plants) to full patent protection for the GM seeds commercialized in the 1990s (Howard 2009; Pardey et al., 2013).
Although earlier plant variety protections allow farmers to save seeds, full patents prohibit this practice and violators may suffer serious financial penalties.
The development of corn (maize) hybrids (which do not breed true if seeds are replanted) initially encouraged the growth of a private corn seed industry, but this biological approach to controlling replanting has been significantly expanded with the legal approach of controlling IPRs through patenting of seeds.
The consolidation in the global seed industry which followed the introduction of GM crops is associated with reduced choices including decreasing access to non-patented (and non-GM) seed varieties for staple crops.
In Europe, public opposition to GM crops began when food products, including unlabelled GM soya for use in processed foods, began to be imported from the USA in 1996.
People raised concerns including: why do we need GMOs; who will benefit from their use; who decided that they should be developed and how; why are we not given an effective choice about whether or not to buy these products; have the potential long-term and irreversible consequences been evaluated, and by whom; do regulatory authorities have sufficient powers to effectively regulate large companies who wish to develop these products; can controls imposed by regulatory authorities be applied effectively; and who will be accountable in cases of unforeseen harm? (Marris 2001).
Seventeen years later, there is still no market for GM foods in Europe and only a small quantity of Bt maize is grown, mainly in Spain, for use in animal feed. However, grain-fed livestock production in Europe is now depend- ent on imported feed, much of which is GM.
Controversy remains about potential unintended effects of GM foods on human health, and the difficulties in assessing such effects using short term animal feeding studies (e.g. Dona & Arvanitoyannis 2009, De Vendômois et al. 2009, Jiao et al. 2010, Aris & LeBlanc 2011).
There are five main areas of food safety concern:
* Whether the genetic modification itself may make the plant toxic when eaten, or alter its nutrient content in ways that may be harmful;
* The presence of herbicide residues on herbicide-tolerant GM crops, and impacts on local populations during spraying;
* Whether the new GM characteris- tic may cause allergies;
* If antibiotic resistance genes are used, whether this will contribute to antibiotic-resistance;
* Whether the GM process has unin- tended effects on the plant, which may affect food safety.
Case-by-case risk assessment is generally required by regulators, but since animal studies cannot reach definitive conclusions, and new traits can always introduce new risks, segregation and labeling of GM crops are an important part of risk management (allowing recalls if anything goes wrong) and are regarded as essential in many countries to allow consumer choice.
Because GM crops generally command a lower market price, for non- GM farmers key issues are cross-contamination and liability (Rogers, 2007).
The costs of segregating GM crops fall on conventional and organic farmers, rather than on those choosing to grow or import GM crops, and thus limit choice for non-GM growers by damaging their markets (Johnson et al. 2005, Belcher et al., 2005, Munro 2008, Binimelis 2008).
A variety of mechanisms, including seed mixing or cross-pollination (Knispel et al. 2008; Schafer et al. 2011) can spread GM traits and in some cases have caused major (multi-million dollar) damage to markets for conventional or organic crops and foods (Smythe et al. 2002).
Farmers and consumers may also choose not to grow or eat GM crops for environmental reasons.
In the UK, the decision not to grow GM crops commercially followed publication of the results of the Farm Scale Evaluations (FSEs), which found that growing herbicide tolerant oilseed rape and sugar beet would be likely to reduce weed food sources and habitats for birds and other wildlife (Squire et al. 2003).
Long-term environmental impacts of growing GM crops remain controversial and poorly understood.
For example, the loss of agricultural milkweeds has contributed to a major decline in the Monarch butterfly population in the USA, coincident with the increased use of glyphosate in conjunction with increased planting of GM glyphosate-tolerant corn (maize) and soybeans (Pleasants & Oberhauser 2013).
Controversy has also surrounded potential impacts of Bt toxins on non-target organisms, such as green lacewing and Monarch butterfly larvae, and there remain important gaps in knowledge (Hilbeck & Schmidt 2006; Lang & Otto 2010).
Next-generation GM crops
Salt-tolerant and nitrogen-fixing crops were first promised by the US Office of Technology Assessment in its 1981 report on genetic engineering of micro-organisms, plants and animals (OTA 1981).
In 2010, experts involved in the UK Foresight ‘Global Food and Farming Futures’ project predicted development timescales of a further 5 to 10 years for GM drought-tolerance, 10 to 20 years for salt-tolerance and increased nitrogen use efficiency, and more than 20 years for nitrogen fixa- tion in GM crops (Godfray et al. 2010).
Some have questioned whether such complex traits can really be engineered into a seed, even over these long timescales: multiple genes and gene- environment interactions are involved and engineering even multiple genetic changes into a plant may not deliver the desired response to multiple environmental stressors (Flowers 2004; Tuberosa & Salvi 2006; Cattivelli et al. 2008; Sinclair & Purcell 2005).
To date, conventional breeding has been much more successful in improving yields in high salinity environments, at much lower cost (Ashraf & Akram, 2009).
Both DuPont and Syngenta have announced the successful development of new conventionally bred drought-tolerant maize hybrids (Berry 2011).
However, Syngenta has stated that it will only market this with two existing GM traits (herbicide tolerance and pesticide resistance), thus allowing it to claim patent protection and market the crop with its own-brand herbicide (Ranii 2010).
In a draft assessment of Monsanto’s new drought-tolerant GM maize the US Department of Agriculture (USDA) has stated that equally comparable varieties produced through conventional breeding techniques are readily available (Voosen 2011).
There are plenty of other non-GM successes, including drought-tolerant non-GM maize which has reportedly performed well in studies in Africa (Cocks 2010). Monsanto has stated that it does not expect new biotechnology traits that contribute significantly to yield to be available for at least another 15 years (Stebbins 2011).
Alternatives
The International Assessment of Agricultural Knowledge, Science and Technology for Development has highlighted the potential for agro-ecological methods to contribute to future agricultural production and dietary diversification without the downsides associated with GM crops (IAASTD 2008), as have a number of other more recent reports (e.g. SCAR 2011).
Research into crop rotation, inter-cropping and other low-input methods has potential to substantially benefit smallholder farmers in Africa and elsewhere (e.g. Fenning et al. 2006, Jeranyama et al. 2007).
At the same time, conventional breeding continues to deliver significant crop improvements in areas where sufficient investment has been made and new technologies such as Marker Assisted Selection (MAS) have demonstrated potential to speed up conventional breeding methods.
Roughly 30 to 40% of food in both the developed and developing worlds is lost to waste (Godfrey et al. 2010). Important steps could be taken to reduce waste by improving storage and transport infrastructure in developing countries and tackling consumer waste elsewhere: this requires alternative investment priorities, for example in engineering (Institute of Mechanical Engineers 2012).
Discussion
The argument that GM crops are needed to feed the world misrepresents hunger as being essentially an issue of insufficient agricultural production, rather than of poverty and unequal distribution of resources (Rosset 2005).
Based on grain consumption figures calculated by the Food and Agriculture Policy Research Institute, Monsanto argues that production of grain for animal feed must increase by 50 mil- lion tonnes a year by 2017/18 to meet the expected increased demand for grain-fed meat, and by 60 million tonnes a year to meet biofuels production targets: requiring more invest- ment in intensive agriculture, including GM crops (Edgerton 2009).
However, the diversion of potential food-growing land to produce industrial-scale biofuels and animal feed is part of the problem, not the solution, to global hunger (FAO 2008). At the same time as claiming GM crops are needed to help feed the world, Monsanto and other companies have been actively lobbying for government subsidies for industrial-scale biofuels (Anon 2008).
Grain-fed meat production is also significantly more resource intensive and damaging to the environment than pasture-fed meat production, as well as less healthy than pasture-fed meat: tackling such issues requires fundamental reform of food and agricultural systems (Lobstein 2004).
Loss of autonomy (i.e. dependence on regulators to assess safety and ensure choice) has been a key element of consumer rejection of GM crops in Europe, and loss of autonomy for farmers (including the prevention of seed saving and dependency on a small number of multinational compa- nies) is a major issue for developing countries.
The advent of patents on GM plants has contributed to the takeovers and mergers which have led to consolidation of the seed industry (King JL & Schimmelpfennig 2005).
This has led in a shift in both public and private research toward the most profitable proprietary crops and varieties and away from the improvement of varieties that farmers can easily replant; and a reduction in seed diversity, as remaining firms eliminate less profitable lines from newly acquired subsidiaries (Howard 2009).
In addition, research into agricultural systems for crop or animal production has received minimal funding, as the knowledge cannot be privatised through patenting (Vanloqueren & Baret 2009).
The patenting system and the reorganisation of research funding systems that goes with it have their origins in the USA in the 1980s and have been widely adopted in OECD countries in an attempt to remain competitive in the face of lower labour costs elsewhere.
It is highly questionable whether this model of innovation and associated economic development has been successful and whether it is an appropriate model to export to developing countries.
Appropriate R&D priorities are more likely to result from engaging local people in decision-making on the ground, bearing in mind that significant opportunity costs can arise from the misallocation of resources.
Conclusions
Promises of a new generation of GM crops, including crops which could grow in saline environments or fix nitrogen, were first made over thirty years ago, by the US Office of Technology Assessment.
Significant public and private investment has been made in GM crop research because GM seeds can be patented, allowing a high return on investment due to the monopoly granted by the ownership of patents.
It is questionable whether a research system driven by the public interest, rather than by monopolistic commercial interests, would have invested so heavily in this technology, when conventional breeding, dietary diversification and agroecological methods have generally proved more effective at delivering complex traits such as drought-tolerance, improved nutrition and increased yields.
A major downside associated with GM crops has been the loss of autonomy for both farmers and consumers, through the prevention of seed saving via patenting and licensing agreements, and the loss of consumer choice and dependence on scientific risk assessments to determine the validity of safety and health claims.
A number of wealthy western institu- tions are currently promoting the expansion of GM crops in developing countries.
A major concern is the potential to create a ‘poverty trap’ for poorer farmers as resistant weeds and pests develop (requiring more expensive inputs and reducing yields) and as companies introduce seed price hikes as they gain monopoly control over the seed market.
A key issue is who decides research priorities for Africa: rich western donors with their own agenda, or farmers on the ground.
References
Anon (2008) Biggies from agribusiness join new biofuels lobbying group. Associated Press. 25th July 2008. http://www.livemint.com/2008/07/25130050/ Biggies-from-agribusiness-join.html
Aris A, LeBlanc S (2011) Maternal and fetal exposure to pesticides associated to genetically modified foods in Eastern Townships of Quebec, Canada. Reproductive Toxicology, 31(4):528-33.
Ashraf M, Akram NA (2009) Improving salinity tolerance of plants through conventional breeding and genetic engineering: an analytical comparison. Biotechnology Advances, 27, 744-752.
Belcher K, Nolan J, Phillips PWB (2005) Genetically modified crops and agricultural landscapes: spatial patterns of contamination. Ecological Economics, 53(3), 387-401.
Benbrook CM (2012) Impacts of genetically engineered crops on pesticide use in the U.S. -- the first sixteen years. Environmental Sciences Europe 24(1):24.
Berry, I (2011) DuPont Wades Into Drought- Tolerant Corn. Wall Street Journal. 5th January 2011. http://online.wsj.com/article/SB100014240527 48704405704576063961961160744.html
Binimelis R (2008) Coexistence of Plants and Coexistence of Farmers: Is an Individual Choice Possible? J Agric Environ Ethics 21(5):437–457.
Binimelis, R, Pengue, W, Monterroso, I (2009) ‘‘Transgenic treadmill”: Responses to the emergence and spread of glyphosate-resistant johnsongrass in Argentina. Geoforum, doi:10.1016/j.geoforum.2009.03.009. http://icta.uab.cat/99_recursos/124176953257 8.pdf
Bonny S (2011) Herbicide-tolerant Transgenic Soybean over 15 Years of Cultivation: Pesticide Use, Weed Resistance, and Some Economic Issues. The Case of the USA. Sustainability, 3(12):1302–1322.
Catangui MA, Berg RK (2006) Western Bean Cutworm, Striacosta albicosta (Smith) (Lepidoptera: Noctuidae), as a Potential Pest of Transgenic Cry1Ab Bacillus thuringiensis Corn Hybrids in South Dakota. Environmental Entomology 35:1439–1452.
Cattivelli L, Rizza F, Badeck F-W, Mazzucotelli E, Mastrangelo AMM, Francia E, Marè C, Tondelli A, Stanca AM (2008) Drought tolerance improvement in crop plants: An integrated view from breeding to genomics. Field Crops Research, 105(1-2), 1-14.
Cocks, T (2010) Drought tolerant maize to hugely benefit Africa: study. Reuters. 25th August 2010.
http://www.reuters.com/article/2010/08/26/us -maize-africa-idUSTRE67P01V20100826
De Schutter, O. (2010) Report submitted by the UN Special Rapporteur on the Right to Food Olivier De Schutter to the UN General Assembly, 17 December 2010.
De Vendômois JS, Roullier F, Cellier D, Séralini G-E (2009) A comparison of the effects of three GM corn varienties on mammalian health. International Journal of Biosciences, 5(7), 706-726.
Dhurua S, Gujar GT (2011) Field-evolved resistance to Bt toxin Cry1Ac in the pink bollworm, Pectinophora gossypiella (Saunders) (Lepidoptera: Gelechiidae), from India. Pest Management Science 67(8):898–903.
Dona A, Arvanitoyannis IS (2009) Health risks of genetically modified foods. Critical Reviews in Food Science and Nutrition, 49, 164-175.
Edgerton MD (2009) Increasing crop productivity to meet global needs for food and fuel. Plant Physiology, 149, 7-13.
FAO(2008) Soaring food prices: facts, perspectives, impacts and actions required. High-level conference on world food security: the challenges of climate change and bioenergy. Food and Agriculture Organisation. Rome, 3 - 5 June 2008. http://www.fao.org/fileadmin/user_upload/foo dclimate/HLCdocs/HLC08-inf-1-E.pdf
Fenning JO, Gyapong TA, Ababio F, Gaisie E (2006) Intercropping maize with planted fallows on smallholder farms in Ghana. Tropical Science, 45(4), 155-158.
Flowers TJ(2004) Improving crop salt tolerance. Journal of Experimental Botany, 55(396), 307-319.
Gassmann AJ, Petzold-Maxwell JL, Keweshan RS, Dunbar MW (2011) Field-Evolved Resistance to Bt Maize by Western Corn Rootworm. PLoS ONE. 6(7):e22629.
Glover, D (2010) Exploring the Resilience of Bt Cotton’s “Pro-Poor Success Story”. Development and Change, 41 (6): 955–981.
Godfray HCJ, Beddington JR, Crute IR, Haddad L, Lawrence D, Muir JF, Pretty J, Robinson S, Thomas SM, Toulmin C (2010) Food security: the challenge of feeding 9 billion people. Science. www.sciencexpress.org / 28 January 2010 / Page 1 / 10.1126/science.1185383
Gunning RV, Dang HT, Kemp FC, Nicholson IC, Moores GD (2005) New resistance mechanism in Helicoverpa armigera threatens transgenic crops expressing Bacillus thuringiensis Cry1Ac toxin. Appl. Environ. Microbiol. 71(5):2558–2563.
Hilbeck A, Schmidt J (2006) Another view on Bt-proteins – how specific are they and what else might they do? Biopesticides Int. 2:1–50.
Howard PH (2009) Visualizing Consolidation in the Global Seed Industry: 1996–2008. Sustainability, 1(4):1266–1287.
IAASTD (2008) International Assessment of Agricultural Knowledge, Science and Technology for Development (IAASTD) global summary for decision makers. http://www.agassessment.org/docs/Global_SD M_060608_English.pdf
Institute of Mechanical Engineers (2013) Global Food: Waste not, want not. London, January 2013.
Jacobsen S-E, Sørensen M, Pedersen SM, Weiner J (2013) Feeding the world: genetically modified crops versus agricultural biodiversity. Agron. Sustain. Dev. DOI 10.1007/s13593-013- 0138-9
Jeranyama P, Waddington SR, Hesterman OB, Harwood RR (2007) Nitrogen effects on maize yield following groundnut in rotation on smallholder farms in sub-humid Zimbabwe.
African Journal of Biotechnology, 6 (13), 1503- 1508.
Jiao Z, Si X-X, li G-K, Zhang Z-M, Xu X-P (2010) Unintended Compositional Changes in Transgenic Rice Seeds (Oryza sativa L.) Studied by Spectral and Chromatographic Analysis Coupled with Chemometrics Methods. Journal of Agricultural and Food Chemistry, 58, 1746–1754.
Johnson DD, Lin W, Vocke G (2005) Economic and welfare impacts of commercializing an herbicide tolerant, biotech wheat. Food Policy, 30, 162-184.
King JL & Schimmelpfennig D (2005) Mergers, acquisitions, and stocks of agricultural biotechnology intellectual property. AgBioForum, 8, 83-88.
Knispel AL, McLachlan SM, Van Acker RC, Friesen LF (2008) Gene Flow and Multiple Herbicide Resistance in Escaped Canola Populations. Weed Science 56(1):72–80.
Lang A, Otto M (2010). A synthesis of laboratory and field studies on the effects of transgenic Bacillus thuringiensis (Bt) maize on non-target Lepidoptera. Entomologia Experimentalis et Applicata 135(2):121–134.
Lobstein T (2004) Suppose we all ate a healthy diet...? Eurohealth, 10(1), 8-12. http://www.sustainweb.org/pdf/afn_m6_p2.pd f
Lu Y, Wu K, Jiang Y, et al. (2010) Mirid Bug Outbreaks in Multiple Crops Correlated with Wide-Scale Adoption of Bt Cotton in China. Science 328(5982):1151–1154.
Marris C (2001) Public views on GMOs: deconstructing the myths. EMBO Reports, 2(7), 545-548.
Meyer G (2011) US ethanol refiners use more corn than farmers. Financial Times. 12th July 2011. http://www.ft.com/cms/s/0/77dfcd98- ac9f-11e0-a2f3- 00144feabdc0.html#axzz1RmTAJ7w3
Munro A (2008) The spatial impact of genetically modified crops Ecological Economics, 67(4), 658-666.
NAS (1987) Agricultural Biotechnology: Strategies for National Competitiveness Committee on a National Strategy for Biotechnology in Agriculture, National Research Council. ISBN: 0-309-59573-8, 224 pages. http://www.nap.edu/catalog.php?record_id=1 005
Oguamanam C (2007) Tension on the farm fields: The death of traditional agriculture? Bulletin of Science, Technology & Society, 27(4), 260-273.
OTA(1981) Impacts of applied genetics: Micro- organisms, plants and animals. US Office of Technology Assessment. April 1981. http://www.fas.org/ota/reports/8115.pdf
Pardey P, Koo B, Drew J, Horwich J, Nottenburg C (2013) The evolving landscape of plant varietal rights in the United States, 1930-2008. Nature Biotechnology 31(1):25–29.
Pleasants JM, Oberhauser KS (2013) Milkweed loss in agricultural fields because of herbicide use: effect on the monarch butterfly population. Insect Conservation and Diversity 6(2):135–144.
Ranii D (2010) Drought-tough corn seed races to the finish line. News Observer. 21st December 2010. http://www.newsobserver.com/2010/12/21/87 3577/drought-tough-corn-seed- races.html#ixzz19bnyr2KL
Rodgers C (2007) Coexistence or conflict? A European perspective on GMOs and the problem of liability. Bulletin of Science, Technology and Society, 27(3), 233-250.
Rosset PM (2005) Transgenic crops to address Third World hunger? A critical analysis. Bulletin of Science, Technology & Society, 25, 306-313.
Sandermann H (2006) Plant biotechnology: ecological case studies on herbicide resistance. Trends in Plant Science, 11(7), 324-328.
SCAR (2011) The 3rd SCAR Foresight Exercise: Sustainable Food Consumption and Production in a Resource-constrained World. European Commission Standing Committee on Agricultural Research, February 2011.
Schafer MG, Ross AA, Londo JP, et al. (2011) The Establishment of Genetically Engineered Canola Populations in the U.S. PLoS ONE 6(10):e25736.
Sinclair TR, Purcell LP (2005) Is a physiological perspective relevant in a ‘genocentric’ age? Journal of Experimental Botany, 56(421), 2777- 2782.
Smyth S, Khachatourians GG, Phillips PWB (2002) Liabilities and economics of transgenic crops. Nat Biotech. 20(6):537–541.
Squire GR, Brooks DR, Bohan DA, et al. (2003) On the rationale and interpretation of the
Farm Scale Evaluations of genetically modified herbicide-tolerant crops. Philos Trans R Soc Lond B Biol Sci. 358(1439):1779–1799.
Stebbins, C (2011) USDA economist: Hard to pin crop number on climate. Reuters. 19th July 2011. http://www.reuters.com/article/2011/07/20/us -climate-agriculture-idUSTRE76J0M320110720
Stone GD (2012) Constructing Facts. Economic and Political Weekly, 47(38):62–70.
Stone, GD, Glover, D (2011) Genetically Modified Crops and the “food Crisis”: Discourse and Material Impacts. Development in Practice 21 (4–5): 509–516.
Tabashnik BE, Gassman AJ, Crowder DW, Carriére Y (2008) Insect resistance to Bt crops: evidence versus theory. Nature Biotechnology, 26, 199 – 202.
Tuberosa R, Salvi S (2006) Genomics-based approaches to improve drought tolerance of crops. Trends in Plant Science, 11(8), 405-412.
Vanloqueren G, Baret PV (2009) How agricultural research systems shape a technological regime that develops genetic
engineering but locks out agroecological innovations. Research Policy 38: 971–983.
Van Rensburg JBJ (2007) First report of field resistance by the stem borer, Busseola fusca (Fuller) to Bt-transgenic maize. South African Journal of Plant and Soil, 24(3):147–151.
Voosen P (2011) USDA Looks to Approve Monsanto's Drought-Tolerant Corn. New York Times. 11th May 2011. http://www.nytimes.com/gwire/2011/05/11/1 1greenwire-usda-looks-to-approve-monsantos- drought-tolera-84634.html
Wan P, Huang Y, Wu H, et al. (2012) Increased Frequency of Pink Bollworm Resistance to Bt Toxin Cry1Ac in China. PLoS ONE. 7(1):e29975.
Wang S, Just DR, Andersen PP (2008) Bt-cotton and secondary pests. International Journal of Biotechnology 10(2/3):113.
Zhao JH, Ho P, Azadi, H (2011) Benefits of Bt cotton counterbalanced by secondary pests? Perceptions of ecological change in China. Environ Monit Assess, 173:985–994.
Download pdf